Microbes
Microbes—the microscopic heroes that make life on this planet possible. As soil scientists discover how microbial life is necessary for healthy soil, as doctors learn how microbial life in our guts is necessary for healthy digestion and immune responses, other researchers learn how bacteria maintain the balance of oxygen on earth.
We can scarcely scratch the surface of this vast topic where new discoveries are made every day, but our aim is to increase awareness of this invisible world that is absolutely necessary for a healthy biosphere.
Soil Food Web is a term we hear more and more often. And for a good reason. Fertile, living soil contains a web of life that creates structure, produces nutrients and controls disease. Almost all soil organisms need carbon-based energy to sustain life. The source of this carbon is mostly organic, such as plant exudates, waste products of microbial metabolism, or the bodies of organisms that are no longer alive. A vast world of microbes, mostly bacteria and fungi, form the base of the soil food web. Here is a simplified depiction of who eats whom and how every component is interdependent. The best way to attain a healthy food web in the soil is to facilitate microbial diversity, which begets the biological diversity which serves that interdependence.
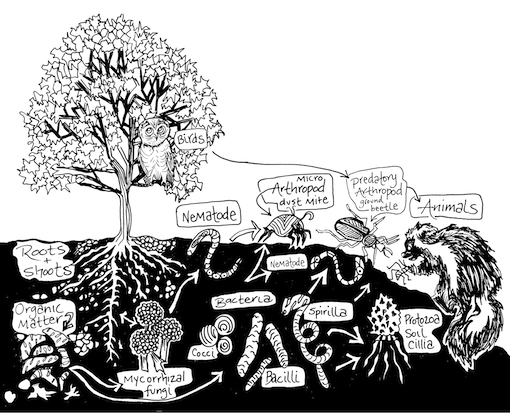
One teaspoon of healthy soil may contain a billion bacteria, several yards of fungal hyphae, thousands of protozoa and dozens of nematodes.
In one acre of topsoil, the top 4" may contain 2000 lb of bacteria, 2400 lb of fungi, 900 lb of algae, 900 lb of earthworms, 133 lb of protozoa.
1 millimeter = 1,000 microns
Bacteria = 1 micron
Fungi = 3 to 5 microns
Nematodes = 5 to 100 microns
Protozoa = 10 to 100 microns
Mites = 100 microns to 5 millimeters
250,000 to 500,000 bacteria can fit inside the period at the end of this sentence.
Microbes make compost. Compost makes soil. If you look at healthy, active compost under a microscope, you’ll see a kaleidoscope of micro-organisms, a majority of them bacteria and fungi. They are hard at work disassembling organic matter from which they extract carbon (C) to fuel their metabolisms, and nitrogen (N) to generate their bodies as well as synthesize the enzymes needed for decomposition. The C:N ratio of the decaying organic material is what distinguishes compost from a pile of rotting stuff. We manipulate the ratio by the mix of ingredients and conditions necessary for the desired microbes to proliferate. On the flip side, subtle fluctuations of this ratio inform yet another ratio, one between the biomass of fungi and bacteria (F:B) in the compost. This ratio, in turn, determines which nitrogen compounds will be abundant in the finished compost, and therefore in the soils that are inoculated/fertilized by it and therefore which plants will thrive in it.
Woody plants such as shrubs and trees generally use nitrogen in the form of ammonium to build their bodies, while most vegetables and grasses use nitrates. Many bacteria, and especially N-fixing kinds, buffer their soil environment at a higher pH than fungi. Since nitrifying bacteria are needed in sufficient numbers to convert ammonium into nitrites and nitrates, most fungally dominated soils are not abundant in nitrates but in ammonium, allowing trees and shrubs to thrive, who in turn release specific root exudates to summon and feed the fungi. On the other hand, annuals, garden vegetables and grasses prefer a lower fungal-to-bacterial biomass ratio because of their preference for nitrates.
Potatoes prefer an even ratio of fungi and bacteria in the soil, or a slightly smaller proportion of fungi to bacteria.
Carrots, salad greens and brassicas prefer between 3–8 parts fungi to 10 parts bacteria.
Bacteria cannot break down lignin, the tough brown component of woody material. Only fungi can decompose lignin by releasing the enzyme phenol oxidase.
One of the major functions of bacteria in soil is decomposition. By releasing myriad enzymes (that magically do not break down their own membranes), bacteria decompose plant and animal matter (cellulose and chitin) into minuscule electrically charged particles that they transport through their cellular membranes. In this way, bacteria ingest nitrogen, carbon compounds and other nutrients that, once ingested, are fixed in their bodies until the bacteria are consumed by other organisms or otherwise die and decay.
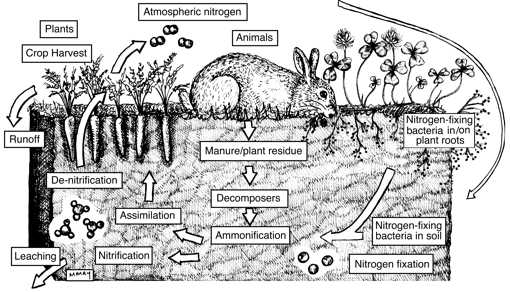
Nitrogen Cycle Not all nitrogen-fixing bacteria are created equal. Some live in water, some in soil, some in both. Some are free-living and some live in symbiosis with certain plants. Some symbionts, called rhizospheric, live on the root tissues of plants, and some, endophytic, live in the cellular spaces of the host plant. Some go back and forth. Some form nodules and some just do it on the fly. But all convert atmospheric nitrogen gas (N2) to plant-available forms—ammonium, nitrites and nitrates—and also back to N2. The bacteria retain the nitrogen in appropriate forms near plant roots, while maintaining a dynamic flow that keeps the cycle healthy. This simplified diagram depicts how nitrogen moves from the atmosphere into the soil, through the bodies of every living organism, back into soil and the atmosphere.
Bacteria are often responsible for breaking down toxins and pollutants in the soil.
One bacterium can produce around 5 billion offspring in 12 hours.
Mycorrhizae are fungal bodies living in or on plant root tissue. The fungi seek out and provide water and nutrients to the roots, who in return feed the fungi with their carbohydrate exudates, which are a significant percentage of the energy produced by photosynthesis.
Mycorrhizal fungi play an especially important role in freeing minerals that are chemically locked up in the soil: especially phosphorus, but also copper, calcium, magnesium, zinc and others.
A by-product of mycorrhizal activity is the production of glomalin, a primary compound that improves soil tilth. In simple terms, glomalin glues the tiny clay particles together into larger aggregates, thereby increasing the amount of large pore space, which in turn creates an ideal environment for roots.
Fungi can grow up to 2.4 millimeters an hour.
Phosphate-solubilizing microbes Phosphorus (P) is the second-most important element after nitrogen (N) for proper plant growth. However, it is almost always chemically and physically bound in the soils. Unless solubilized by bacteria or fungi, or even algae, phosphates stay unavailable to plants. Although bacteria also solubilize phosphates, fungi account for a greater percentage of the bioavailable phosphorus in the soil. Some examples of phosphate-solubilizing microbes are aspergillus, penicillium and trichoderma fungi, as well as bacterial genera such as bacillus, pseudomonas, enterobacter, azotobacter, and even some rhizobium species such as Rhizobium leguminosarum normally known for their N-fixing skills.
Battle of the Bugs In addition to deformed frogs, thin eggshells, and emasculated fish, toxic and persistent synthetic pesticides have another—less obvious but equally important—side effect: their impact on the microbial life in the soil. For example, the microorganisms responsible for solubilizing phosphorus and fixing atmospheric nitrogen are less active in pesticide-contaminated soils, and some pesticides disturb the molecular interactions between plant roots and their associated rhizobial colonies. In contrast, microbial pesticides are extremely selective in their action (often effective on only one or two species of insect) and break down quickly in the natural environment. However, they work at the speed of life, not the speed of light, relying on the slow colonization of the plant surface or root zone rather than on the quick knock-down effect of isolated chemicals.
Nitrosomonas and Nitrobacter are soil bacteria that play a major role in nitrogen cycling. Unlike plants, which use light as an energy source, they are chemoautotrophs, which means they get energy from chemical reactions, here converting ammonium and oxygen to nitrites, and nitrites and oxygen to nitrates, while harvesting the energy released by these reactions. Motile bacteria that move through the soil by flailing their flagella, they do not live in symbiotic relationships with plants. Nitrosomonas, in particular, teams up with other microbes, forming clumps to block sunlight, which they cannot tolerate.
All living organisms need sulfur. Sulfate-reducing bacteria reduce sulfate to hydrogen sulfide by oxidizing hydrogen molecules to obtain energy. They are predominantly anaerobes and breathe sulfates. This metabolic activity is essential to the elemental sulfur cycle of the earth. Desulfovibrio vulgaris is an example of sulfur-reducing bacteria.
Tomato mosaic virus (ToMV) is a troublesome virus infecting tomatoes, potatoes and their relatives, and a few other plants. Symptoms include blistering, distortion and mottling of leaves, with overall stunting and poor yields. Like its close relative tobacco mosaic virus (TMV) ToMV usually enters plants during handling, and can spread on a variety of surfaces such as fingers, clothing, tools and other equipment.
Rust fungus, an obligate parasite, does not usually kill its host plant but can seriously impede its growth. Its spores are airborne and germinate on the leaves of the host plant where they settle. Depicted here is wheat rust, under the microscope.
A remarkable function of fungi in soil is manifested through a couple of different symbiotic relationships, one with green algae and the other with plant roots. In both cases fungi get food from the symbiotic partners’ photosynthetic powers. Lichens are green algae living with fungal structures. While the fungi release chemicals to break down rock and wood, the algae make energy out of sunlight.
Cyanobacteria
50% of the oxygen in the atmosphere is released by cyanobacteria, formerly known as blue-green algae. One of the most ancient living organisms. They live in all imaginable habitats. They are photoautotrophs, meaning they derive their energy directly from the sun through photsynthesis and do not depend on any organic material to survive. Cyanobacteria exhale oxygen and also fix nitrogen.
Root exudates are the favorite foods of the major soil bacteria and fungi. They seek the exudates and end up colonizing in large numbers around the rhizosphere where they form a base for the soil food web.
95% of all plants have mycorrhizal relationships.
The effective surface area of a tree root can be increased by 1000 times with mycorrhizal associations.
Microbes and Gaia
Life belongs to bacteria. They permeate the planet and define the biosphere. From the basalt layers deep beneath the ocean floor to the upper reaches of the atmosphere, and even outer space, they are everywhere.
The most ancient life, appearing on the planet shortly after its formation, bacteria have ceaselessly inhabited the Earth for more than 3.5 billion years. Pioneering all major innovations of life, such as death, motility (swimming), sex (gene exchange), photosynthesis (autotrophy) and, more important, symbiosis and symbiogenesis, which is a principal driving force of evolution, leading to myriad life forms, ever more complex and ever more inextricably intertwined. The history of life is one of bacteria; you can “feel” this history even in your own mitochondria!
What’s more is that the metabolic activities of bacteria transform and cycle all earthly elements, and thereby manipulate the geochemical composition of the earth, including its atmosphere. In fact, the early forms of photosynthetic bacteria were responsible for changing the earth’s atmosphere from a stable, 95% CO2 environment to a dynamic, 20% oxygen one which has maintained an anomalous dynamic balance for the last 500 million years. Chemically anomalous because free oxygen is extremely reactive in the presence of other gases. All plant and animal life depends on and helps maintain this atmospheric oxygen level, and the consequent temperature range. A few percentage points less or more would poison most animals or set fire to the forests.
Just as they transformed the atmosphere, bacteria also initiated the creation of the soils in which plants and terrestrial animals evolved. Coupled with fungi, bacteria prepared the early soils of the earth by trapping gaseous carbon, sulfur, nitrogen, and other elements in their bodies, depositing them as various complex compounds, while mixing in all manner of minerals from rocks they “eat.” Together fungi and bacteria formed the foundations of the soil food web. Microbes make all major element cycles possible, allowing all other life forms to proliferate, and transforming earth into an ever-fertile medium for life.
Bacteria as ancestors. Ancestors as teachers.
As we unravel relationships between organic and inorganic stuff of this blue planet, we increasingly understand that the biota and the earthly environment blend into a single entity—a self-organized system with endless feedback loops pulsating back and forth in sentient resilience. The late Lynn Margulis pointed out that, aside from confirming the intuitive human sentiment of “all is one,” such an understanding might suggest “a strong biogeological precedent for the time-honored political and mystical goal of peaceful co-existence and world unity.” Undoubtedly, bacteria will improvise on life until the Sun boils the oceans off of the earth, or all is lost to dark matter. Until then, let us learn from the bacteria as we stand in awe at the balance they masterfully strike.
Fungi as Gaia’s nervous system
All plants are part fungi. Some would argue that plants are merely another form of the fungal way of life: think of roots as mycelium. Although fungal forms have evolved to live in all corners of the biosphere, it is on land where they practice their most elegant crafts: soil making and networking. As Paul Stamets puts it, fungi are the molecular disassemblers of the earth, producing countless acids and enzymes that can break down everything from lignin to cellulose, from hydrocarbons to plain rocks. These acids and enzymes are not haphazardly produced and released into the environment for the sole purpose of exploiting a possible food source, but are vehicles of a chemical communication amongst the fungi, their neighboring organisms, their physical environment and ultimately, their entire eco-range. The ability to travel through the soil, even in the absence of water, allows soil fungi to form cross-bridging networks of unmatched complexity. Mycelium seek and transport not only nutrients, but also information and stories about everything that is in or on the soil and so create an externalized neurological system that serves integrated communities of soil life in the most efficient, decentralized manner.
Like animals, fungi breathe oxygen. That trait identifies and complements fungi’s ubiquitous mastery of symbiotic relationships and symbiogenesis. Although you can find them in foods like kefir and kombucha, or cultivated in subterranean gardens by termites, fungi are most extensively associated with photosynthesizers, such as algae (lichens) and plants (mycorrhizae). Mycorrhizal associations are as ancient as the first plants that appeared on earth and are so embedded in plant life that up to 20% of the carbohydrates produced through photosynthesis by a host plant gets relocated to its symbiont fungal bodies. John Muir said, “When one tugs at a single thing in nature, he finds it attached to the rest of the world.” His observation could not be more accurate as it relates to the activities of fungi in earthly soils.
Microbes
We would like to draw special attention to products that contain or promote microbial life:Microbes:
- Legume Inoculants (Rhizobium spp.)
- Myco Seed Treatment (Endo- and ectomycorrhizal fungi)
- MycoApply All Purpose (Endo- and ectomycorrhizal fungi)
- EM-1 (blend of beneficial microbes)
- Monster Plant Mojo
- Cease (Bacillus subtilis)
- Milky Spore (Bacillus popilliae)
- DiPel (Bacillus thuringiensis)
- Safer Caterpillar Killer (Bacillus thuringiensis)
- Monterey Garden Spray (fermentation product of Saccharopolyspora spinosa)
Aktan Askin provided much of the research, writing, and passion for this project. He used especially the writings of the late Lynn Margulis, Mycelium Running by Paul Stamets, Soil Biology Primer by Elaine Ingham, Teaming with Microbes by Jeff Lowenfels and Wayne Lewis and Plant Intelligence and the Imaginal Realm by Stephen Harrod Buhner.